Eratosthenes first measured the circumference of the earth from the shadows cast by the sun. Today, humanity's fitness to survive will be measured by our ability to conquer that same thermonuclear fusion that casts those shadows. Thus, Prometheus will truly be unbound.
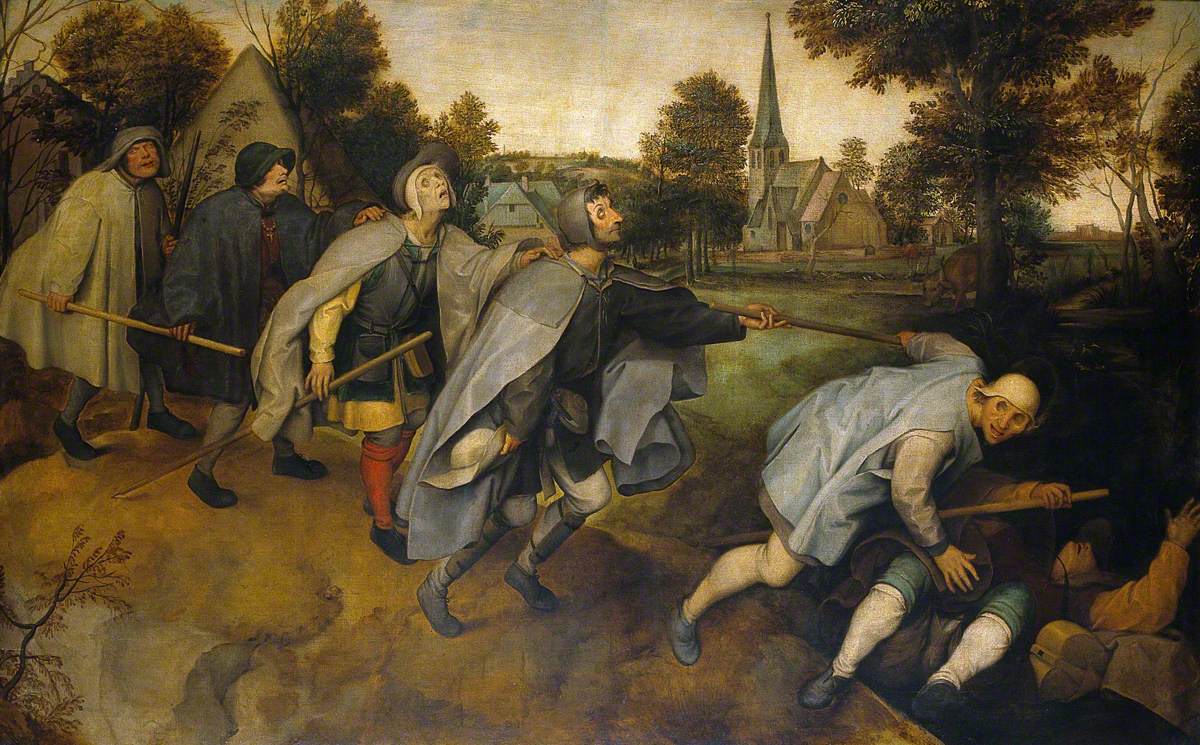
Today's Elites
Friday, November 27, 2009
Riemann surface and biological functions
The Riemann surface models a complex function that has multiple values on sheets connected through functional branch points. Compare that with this functional diagram of the simplest of protein life forms and once again it is clear that Riemann's geometric epistemology was prescient.
This image represents the integration of genomic, metabolic, proteomic, structural and cellular information about Mycoplasma pnemoniae in this project: one layer of an Electron Tomography scan of a bottle-shaped M. pneumoniae cell (grey) is overlaid with a schematic representation of this bacterium's metabolism, comprising 189 enzymatic reactions, where blue indicates interactions between proteins encoded in genes from the same functional unit. Apart from these expected interactions, the scientists found that, surprisingly, many proteins are multifunctional. For instance, there were various unexpected physical interactions (yellow lines) between proteins and the subunits that form the ribosome, which is depicted as an Electron microscopy image (yellow). Credit: Takuji Yamada /EMBL
Thursday, November 26, 2009
A Biophysical Analog of a Higher Order Process Embedded in Apparently Random Mutations
As I pointed to in an earlier post, Riemann's mathematical method in approaching "chaotic" disordered functions through elimination by degree of arbitrariness should prove instructive in applications for applied sciences. Now a new microbiology discovery bears this idea out:
Apparent errors/mutations in RNA translation of protein production lead to extra methionine amino acid randomly being attached to proteins. But this is now shown to be purposeful because these "misreadings" of the protein genetic code lead to protection against reactive oxygen species when cells are under attack by disease or stress and these errors are then greatly accelerated. So, the question that this begs is what is the higher order functional mechanism that directs this apparent disorder? This is precisely where the zenith of scientific epistemology exemplified by nineteenth century Riemann's radically constructive non Euclidean approach needs to be brought to bear.
When cells are confronted with an invading virus or bacteria or exposed to an irritating chemical, they protect themselves by going off their DNA recipe and inserting the wrong amino acid into new proteins to defend them against damage, scientists have discovered.
These "regulated errors" comprise a novel non-genetic mechanism by which cells can rapidly make important proteins more resistant to attack when stressed, said Tao Pan, Professor of Biochemistry and Molecular Biology at the University of Chicago. A team of 18 scientists from the University of Chicago and the National Institute of Allergy and Infectious Disease led by Pan and Jonathan Yewdell published the findings Thursday in the journal Nature.
"This mechanism allows every protein to get some protection," Pan said. "The genetic code is considered untouchable, but this is a non-genetic strategy used in cells to create a bodyguard for proteins."
Proteins are constructed through a process called translation where cellular elements use the genetic code to guide the assembly of building blocks called amino acids into the correct sequence. First, a copy of the DNA, called messenger RNA, is made and transferred to a cellular structure called a ribosome. Transfer RNAs (tRNA), one for each of the 20 amino acids used in building proteins, read the messenger RNA code and bring the proper amino acids to the ribosome, where they are bonded together to form a complete protein.
Each tRNA can be attached to only one of 20 amino acids, a specificity that prevents errors during the construction of proteins. In artificial laboratory preparations, scientists have observed that only one out of every 10,000 amino acids is placed into a protein incorrectly, and thus protein errors were thought to be exceptionally rare.
But Jeffrey Goodenbour, University of Chicago graduate student and co-lead author along with Nir Netzer of the NIAID, decided to look at how often tRNA errors, called misacylations, occurred in live cells. After developing a novel technique for measuring these errors, published for the first time in this paper, the authors were surprised to find a much higher error rate in those cells for the amino acid methionine. As high as one out of every 100 methionines was incorrectly placed in proteins, they found.
When the cells were stressed by exposure to a virus, bacteria or a toxic chemical such as hydrogen peroxide, that error rate went even higher, as up to 10 percent of methionines placed into new proteins were different from what the gene specified.
"That was 1,000 times more than the textbook says should be there," Pan said.
Further experiments revealed that it was always the same amino acid, methionine, placed incorrectly into new proteins. Methionine is one of only two amino acids to carry sulfur atoms on its side chains, a feature that allows it to neutralize dangerous molecules called reactive oxygen species (ROS) that form inside an infected or stressed cell. ROS can damage proteins through a chemical process called oxidation, but methionine can be oxidized (and restored through a process called reduction) without being permanently damaged.
"The idea is that methionine can protect you from having oxidation of the active site of protein, which would ultimately completely block function of the protein," Goodenbour said. "You end up reducing the total reactive oxygen species load in the cell. It's a very interesting mechanism."
Cells normally put methionines near important parts of a protein to protect those segments from being damaged by reactive oxygen species. When the cell is under stress, and the amount of ROS increases, the number of methionine "errors" is ramped up tenfold, allowing new proteins to be even more resistant to attack.
"Think of a boxing match," Pan said. "If you put methionine close to active site, the reactive oxygen species has to get past it to get to the active site residues for oxidization. You've put something right in front of it so a protein can take a hit. If you have a lot of methionines, to knock this protein out will take many, many hits. So this is a strategy used in cells to create a bodyguard for a protein."
A remaining puzzle is to determine why extra protective methionines are not encoded as part of the DNA in the first place, instead of being left to the post-genetic random placement described in this paper. Pan suggests that random placement of the amino acids makes proteins even more resistant to attack, since no two are created alike.
"This sounds chaotic and doesn't make a lot of sense according to the textbook," Pan said. "But this way the cells can always ensure that a subset of these proteins is somewhat less sensitive to the extra hits. I think that's the most important part of this - to make every protein molecule different - and you cannot do this genetically."
Source: University of Chicago Medical Center
Apparent errors/mutations in RNA translation of protein production lead to extra methionine amino acid randomly being attached to proteins. But this is now shown to be purposeful because these "misreadings" of the protein genetic code lead to protection against reactive oxygen species when cells are under attack by disease or stress and these errors are then greatly accelerated. So, the question that this begs is what is the higher order functional mechanism that directs this apparent disorder? This is precisely where the zenith of scientific epistemology exemplified by nineteenth century Riemann's radically constructive non Euclidean approach needs to be brought to bear.
Friday, November 20, 2009
Fast and slow brain waves as analogue carriers versus digital information model of brain
My last entry on Fechner's concept of non interfering waves in reminiscences has gotten an interesting empirical support from new research:
This further refutes the nonsensical delirium that the human mind could ever be modeled as a complex "digital" system. Anyone who has ever experienced the nonlinear leap of creative insight best exemplified in Beethoven's compositions, immediately recognizes the absurdity of such misguided behaviorist assumptions about truly human thought.
ScienceDaily (Nov. 19, 2009) — The human brain is bombarded with all kinds of information, from the memory of last night's delicious dinner to the instructions from your boss at your morning meeting. But how do you "tune in" to just one thought or idea and ignore all the rest of what is going on around you, until it comes time to think of something else? Researchers at the Kavli Institute for Systems Neuroscience and Centre for the Biology of Memory at the Norwegian University of Science and Technology (NTNU) have discovered a mechanism that the brain uses to filter out distracting thoughts to focus on a single bit of information. Their results are reported in 19 November issue of Nature.
Think of your brain like a radio: You're turning the knob to find your favourite station, but the knob jams, and you're stuck listening to something that's in between stations. It's a frustrating combination that makes it quite hard to get an update on swine flu while a Michael Jackson song wavers in and out. Staying on the right frequency is the only way to really hear what you're after. In much the same way, the brain's nerve cells are able to "tune in" to the right station to get exactly the information they need, says researcher Laura Colgin, who was the paper's first author. "Just like radio stations play songs and news on different frequencies, the brain uses different frequencies of waves to send different kinds of information," she says.
Gamma waves as information carriers
Colgin and her colleagues measured brain waves in rats, in three different parts of the hippocampus, which is a key memory center in the brain. While listening in on the rat brain wave transmissions, the researchers started to realize that there might be something more to a specific sub-set of brain waves, called gamma waves. Researchers have thought these waves are linked to the formation of consciousness, but no one really knew why their frequency differed so much from one region to another and from one moment to the next.
Information is carried on top of gamma waves, just like songs are carried by radio waves. These "carrier waves" transmit information from one brain region to another. "We found that there are slow gamma waves and fast gamma waves coming from different brain areas, just like radio stations transmit on different frequencies," she says.
You really can "be on the same wavelength"
"You know how when you feel like you really connect with someone, you say you are on the same wavelength? When brain cells want to connect with each other, they synchronize their activity," Colgin explains. "The cells literally tune into each other's wavelength. We investigated how gamma waves in particular were involved in communication across cell groups in the hippocampus. What we found could be described as a radio-like system inside the brain. The lower frequencies are used to transmit memories of past experiences, and the higher frequencies are used to convey what is happening where you are right now."
If you think of the example of the jammed radio, the way to hear what you want out of the messy signals would be to listen really hard for the latest news while trying to filter out the unwanted music. The hippocampus does this more efficiently. It simply tunes in to the right frequency to get the station it wants. As the cells tune into the station they're after, they are actually able to filter out the other station at the same time, because its signal is being transmitted on a different frequency.
The switch
"The cells can rapidly switch their activity to tune in to the slow waves or the fast waves," Colgin says, "but it seems as though they cannot listen to both at the exact same time. This is like when you are listening to your radio and you tune in to a frequency that is midway between two stations- you can't understand anything- it's just noise." In this way, the brain cells can distinguish between an internal world of memories and a person's current experiences. If the messages were carried on the same frequency, our perceptions of the world might be completely confused. "Your current perceptions of a place would get mixed up with your memories of how the place used to be," Colgin says.
The cells that tune into different wavelengths work like a switch, or rather, like zapping between radio stations that are already programmed into your radio. The cells can switch back and forth between different channels several times per second. The switch allows the cells to attend to one piece at a time, sorting out what's on your mind from what's happening and where you are at any point in time. The researchers believe this is an underlying principle for how information is handled throughout the brain.
"This switch mechanism points to superfast routing as a general mode of information handling in the brain," says Edvard Moser, Kavli Institute for Systems Neuroscience director. "The classical view has been that signaling inside the brain is hardwired, subject to changes caused by modification of connections between neurons. Our results suggest that the brain is a lot more flexible. Among the thousands of inputs to a given brain cell, the cell can choose to listen to some and ignore the rest and the selection of inputs is changing all the time. We believe that the gamma switch is a general principle of the brain, employed throughout the brain to enhance interregional communication."
Can a switch malfunction explain schizophrenia?
People who are schizophrenic have problems keeping these brain signals straight. They cannot tell, for example, if they are listening to voices from people who are present or if the voices are from the memory of a movie they have seen. "We cannot tell for sure if it is this switch that is malfunctioning, but we do know that gamma waves are abnormal in schizophrenic patients," Colgin says. "Schizophrenics' perceptions of the world around them are mixed up, like a radio stuck between stations."
This further refutes the nonsensical delirium that the human mind could ever be modeled as a complex "digital" system. Anyone who has ever experienced the nonlinear leap of creative insight best exemplified in Beethoven's compositions, immediately recognizes the absurdity of such misguided behaviorist assumptions about truly human thought.
Subscribe to:
Posts (Atom)